UHS deliverability depends on several factors: (1) H2 energy content per volume of rock occupied by H2/CH4 working gas, (2) sweep efficiency, which is how much of the storage zone is occupied by H2/CH4 working gas, (3) well injectivity and (4) productivity, possibly degraded by (5) microbial and geochemical mechanisms, and (6) asset loss. These factors are influenced by the following:
- Engineering decisions: H2 and CH4 volume fractions in the working gas, storage depth (affecting storage pressure), and injection rate (affecting viscous fingering) of the H2/CH4
- Fluid properties of H2, CH4, and formation brine: mass density, viscosity, and diffusivity.
- Storage formation properties: permeability, porosity, wettability, and permeability heterogeneity.
The H2 energy content per volume of rock (MJ/m3) occupied by the working H2/CH4 gas mixture,
, is determined by the following equation:

where
is mass density (kg/m3) of H2 at temperature and pressure,
is lower heating value (120 MJ/kg) of H2,
is volume fraction of H2 in the gas phase for the working gas,
is porosity, and
is irreducible water saturation. The CH4 energy content per volume of rock,
, is given by a similar equation, with subscript H2 replaced by CH4 and where
is 50 MJ/kg. The total energy content per volume of rock is equal to the sum of
and
. The large mass-density contrast between H2 and CH4 and the fact that mass and volumetric energy densities of H2 and CH4 increase nearly linearly with pressure (Figure 5.1-1a) need to be accounted for in making key engineering decisions, such as the volume fractions of H2 and CH4 in the working gas and storage depth. Increasing the volumetric fraction of H2 increases H2 energy content; however, it also increases the buoyancy driving force that governs gravity override (Figure 5.1-1b), which may reduce sweep efficiency and cause more stranding of H2/CH4 working gas. Compared to 100% H2, an 80/20 H2/CH4 mixture increases total energy density by a factor of 1.6, reduces H2 energy content by 20%, while reducing the buoyancy driving force by a factor of 3.5. Compared to 100% H2, a 50/50 H2/CH4 mixture increases total energy density by a factor of 2.5, reduces H2 content by 50%, while reducing the buoyancy driving force by a factor of 5.2. Compared to 100% H2, a 20/80 H2/CH4 mixture increases total energy density by a factor of 3.4, reduces H2 content by 80%, while reducing the buoyancy driving force by a factor of 7.8. The determination of optimal H2/CH4 mixtures will require reservoir analyses that assess the tradeoff between volumetric energy density and the impact of buoyancy on sweep efficiency and storage-formation utilization.
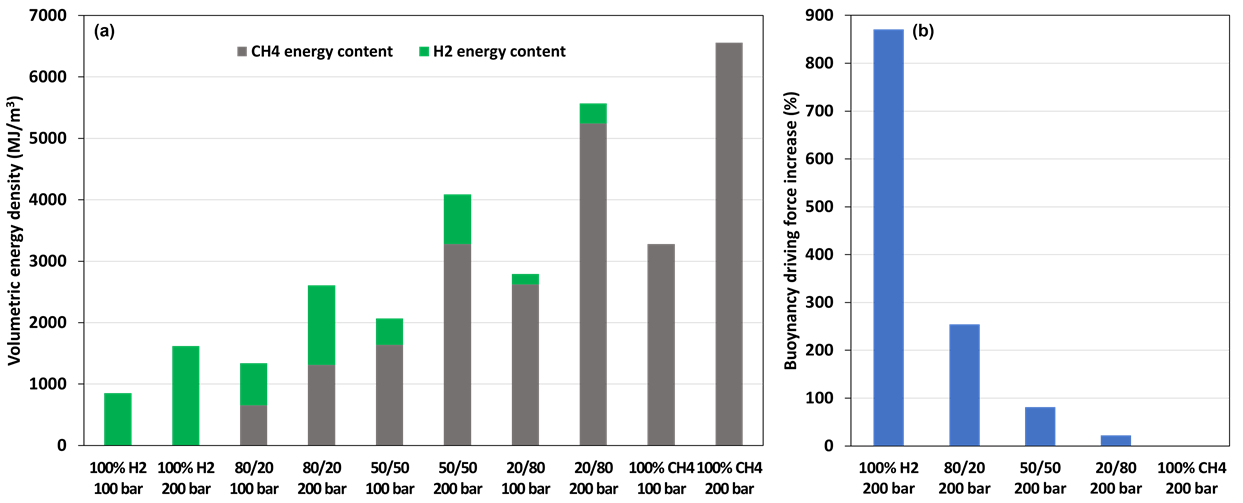
Figure 1. Volumetric energy density of H2/CH4 mixtures for pressures of 100 and 200 bar and a temperature of 50 °C (a) and buoyancy driving force increase compared to 100% CH4 for 200 bar and 50 °C (b). The increase in buoyancy driving force is similar for 100 and 200 bar. Note that the H2/CH4 mixtures are on a per volume basis.